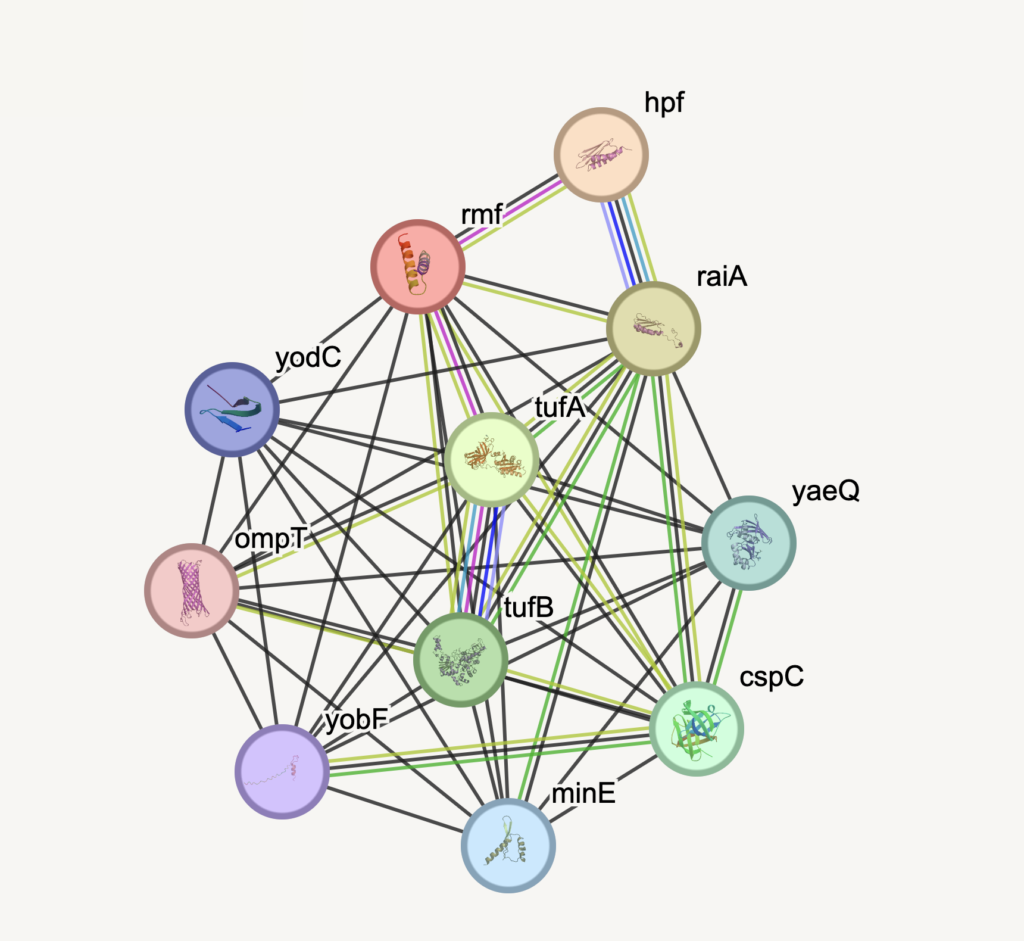
How did plants emerge on our planet? Fossil evidence suggests that the first plant-type organisms initially emerged in the form of algae, likely around 1.2 billion years ago. And for more than 700 million years, they remained exclusively marine, thriving while submerged in water (possibly following the famous crab’s advice: “Darling, it’s better down where it’s wetter”). This is especially remarkable because current evidence indicates that the first masses of land emerged above sea level about 3.7 billion years ago. This means that for over 3 billion years, land was completely devoid of plants. However, about 470 million years ago, marine plants began to transition to terrestrial living, with ferns being among the pioneers of these early conquerors of the land.
We can imagine that terrestrial living provided plenty of benefits to these early plants: little competition for space, much more sunlight, and access to mineral nutrients. Perhaps there were also fewer predators. However, with these advantages came the real risk of death from dehydration, which likely promoted the selection of plant variants capable of tolerating prolonged lack of water. It took some lineages of plants a gradual evolution into seed plants around 350 million years ago during the Late Devonian period. The invention of seeds has provided a colossal advantage for plant survival. For many higher plants, seeds can lose up to 95% of their water content and still remain viable, entering a state of dormancy that protects them from desiccation damage. This allows plants to pause their life cycle until the environment is suitable for active growth.
According to current estimates, the emergence of seed plants coincided with (or preceded by) the origin of so called LEA proteins, which is a special class of protective proteins in plant genomes: . These proteins, through a poorly understood mechanism, allow seeds to remain viable in the face of desiccation. We currently know that most known LEA proteins are highly hydrophilic and often non-globular proteins that protect seeds, as well as mature plant tissues, from desiccation damage. Their name stands for Late Embryogenesis Abundant proteins, which stems from their discovery in cotton seeds, where LEA proteins accumulate during the late stages of embryo development. In plants, LEA proteins enable organisms to survive severe and reversible dehydration, a phenomenon termed “anhydrobiosis.” Furthermore, the ability of LEA proteins can be replicated in other organisms. For example, LEA proteins can confer desiccation tolerance when recombinantly expressed in mammalian cells. In one particular study, mammalian skin cells were shown to become almost completely nonviable (with only 1% of cells alive) after 4 hours of air drying. However, more than 58% of these cells survived if they expressed the protein dehydrin from maize, the cold acclimation protein WCOR410 from wheat, and LEA protein group 3 from brine shrimp.
The evolutionary origin of LEA proteins in nature has likely been driven by the spread of plants from marine to terrestrial environments, which occurred around 470 million years ago during the Ordovician period. As plants transitioned to land, they faced the risk of desiccation and gradually evolved into seed plants, around 350 million years ago during the Late Devonian period. According to current estimates, it is during this time that LEA proteins emerged as small, highly hydrophilic proteins that protect seeds (but also mature plant tissues) from desiccation damage.
Although “canonical” LEA proteins originate from plants, they appear to have spread to other species via horizontal gene transfer. As a result, LEA are present not only in plants but also in other eukaryotes and many bacteria. In tested species, LEA proteins help cells survive harsh environmental conditions, such as desiccation, cold shock, and possibly many other unfavorable circumstances.
In E. coli, there are at least five LEA proteins, but we understand the biology of just one of them, the RMF protein. This protein binds to ribosomes in metabolically inactive (stationary or transiently stressed) cells and prevents ribosomes from being degraded by cellular nucleases and proteases. This allows E. coli to remain inactive with a lower risk of digesting their essential molecular machinery, making it possible for these bacteria to survive prolonged states of metabolic inactivity and recover when conditions improve or when the cells adapt to a new environment.